- The process of creating a drug - May 23, 2023
- How do scientists model mental disorders in animals? - May 23, 2023
Abstract
Indole, with the chemical formula C8H7N, is a remarkable aromatic and heterocyclic organic compound. Its unique structure features a fused combination of a six-membered benzene ring and a five-membered pyrrole ring. The advancement of indole chemistry can be attributed to the widespread use of the renowned indigo dye. In 1886, Baeyer successfully derived indole from indigo by employing isatin and oxindole, and he further elucidated its structural composition. Indole is naturally present in various sources, including the high-boiling fraction of coal tar, jasmine oil, diverse floral species, pitch, and even in fecal matter, typically in concentrations of up to 2.5%.
This comprehensive article provides a detailed overview of indole, covering its general information, physico-chemical properties, an exploration of its biofunctions, tests for indole detection, chemical properties, synthesis methods, a conclusive summary, and a bibliography.
General Information About Indole [1-6]
Other synonyms names of Indole are: 2,3-Benzopyrrole; 1-Benzazole; Ketole; 1-Azaindene; Benzopyrrole; 2,3-Benzopyrole
IUPAC Name of Indole: 1H-indole
CAS number is 120-72-9
Physico-Chemical Properties of Indole [1-6]
- Molecular Formula C8H7N
- Molar Weight 117.15 g/mol
- Boiling point 254-254 °C
- Melting Point 52-52.5 °C
- Solubility: hot water and 0.36 g/100 g (at 25 °C); hot alcohols (35.9 g/100 g ethanol at 20 °C); ether; benzene; toluene; naphtha; Soluble in fixed oils; propylene glycol; insoluble in mineral oil and glycerol.
- Color/Form: Colorless to yellowish scales, turning red on exposure to light and air.
- Odor: Unpleasant odor at high concentration, odor becomes floral at higher dilutions
Structural formula present on Figure 1.
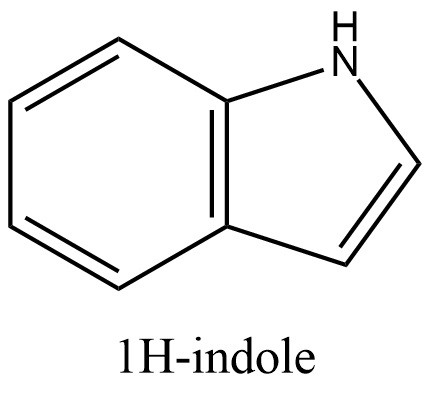
Crystalline solid of the Indole can be seen in the picture provided in Figure 2.
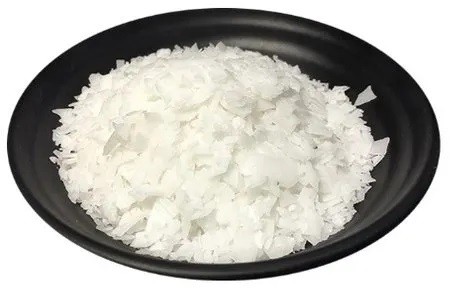
General Chemical Information of Indole [7-9]
This compound falls under the category of organic substances known as indoles. Indoles are compounds that contain an indole component, which is composed of a pyrrole ring fused to a benzene ring, resulting in the formation of 2,3-benzopyrrole. Indole, with the chemical formula C8H7N, is an aromatic and heterocyclic organic compound. It possesses a bicyclic structure, comprising a six-membered benzene ring fused to a five-membered pyrrole ring. Indole is widely distributed in the natural environment and can be synthesized by various bacteria. As an intercellular signaling molecule, indole regulates numerous aspects of bacterial physiology, including spore formation, plasmid stability, drug resistance, biofilm formation, and virulence. Tryptophan, an amino acid, is an indole derivative and serves as the precursor for the neurotransmitter serotonin.
Indole exists as a solid at room temperature. It occurs naturally in human feces, emitting a distinct fecal odor. However, at very low concentrations, it exhibits a floral fragrance and is utilized as a constituent in many perfumes. It is also found in coal tar.
The corresponding substituent for indole is referred to as indolyl. Indole undergoes electrophilic substitution, primarily at position 3. Substituted indoles serve as structural components of tryptophan-derived tryptamine alkaloids, including serotonin (a neurotransmitter), melatonin (a hormone), dimethyltryptamine, and psilocybin (naturally occurring psychedelic drugs). Other indolic compounds include the plant hormone auxin (indolyl-3-acetic acid, IAA), tryptophol, the anti-inflammatory drug indomethacin, and the beta-blocker pindolol.
The name “indole” is a combination of the terms “indigo” and “oleum” since indole was first isolated by treating indigo dye with oleum.
The study of indole chemistry originated from the investigation of the dye indigo. Indigo can be transformed into isatin and subsequently into oxindole. In 1866, Adolf von Baeyer reduced oxindole to indole using zinc dust. In 1869, he proposed a formula for indole.
Certain derivatives of indole played a crucial role as dyes until the late 19th century. In the 1930s, interest in indole grew when it was discovered that the indole substituent is present in many significant alkaloids, known as indole alkaloids (such as tryptophan and auxins). Today, indole chemistry remains an active area of research.
Biofunction of Indole
Indole is synthesized through the shikimate pathway using anthranilate. It serves as an intermediate in the biosynthesis of tryptophan, where it remains within the tryptophan synthase molecule after the removal of 3-phospho-glyceraldehyde and before the condensation with serine. When the cell requires indole, it is typically produced from tryptophan through the action of tryptophanase.
As an intercellular signaling molecule, indole plays a regulatory role in various aspects of bacterial physiology, encompassing spore formation, plasmid stability, drug resistance, biofilm formation, and virulence. Numerous derivatives of indole hold significant cellular functions, including functioning as neurotransmitters like serotonin.
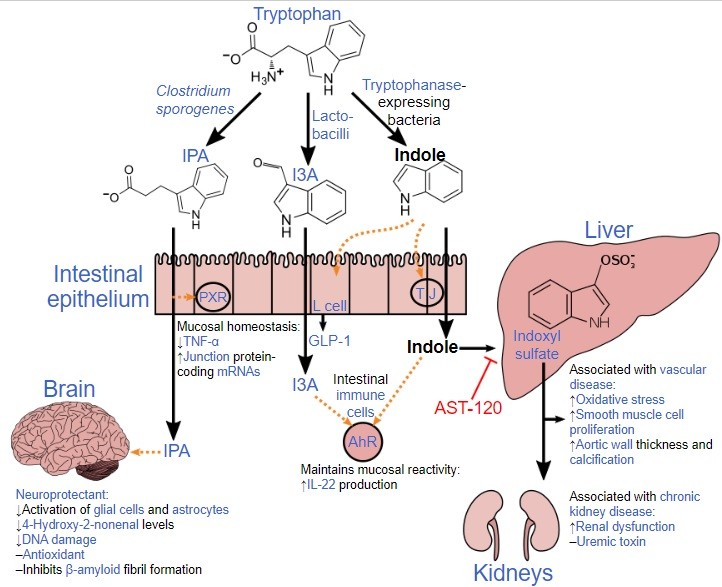
Tests for Indole [9]
Ehrlich’s Test Figure 4
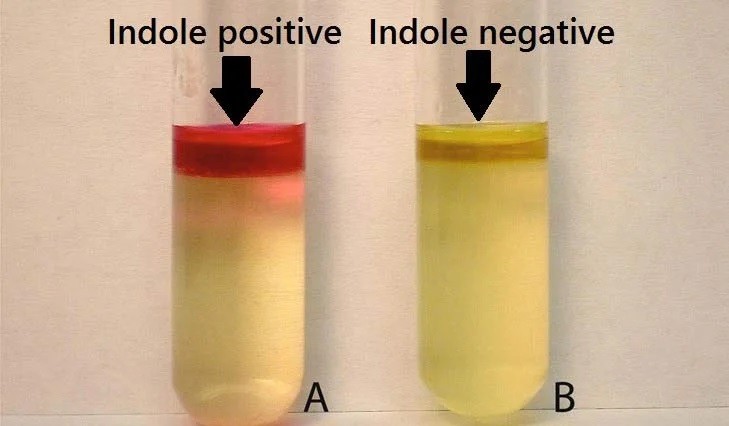
This test illustrates the capability of certain bacteria to break down the amino acid tryptophane into indole, which accumulates in the medium. The production of indole serves as a significant criterion in the identification of Enterobacteria. Most strains of E. coli, P. vulgaris, P. rettgeri, M. morgani, and Providencia species possess the enzymatic machinery to degrade tryptophane, resulting in the release of indole. This process involves a cascade of intracellular enzymes collectively known as “tryptophanase.” The test is commonly employed as part of the IMViC procedures, designed to differentiate members of the Enterobacteriaceae family.
In the case of non-fermenters and anaerobes, a modified version of the test utilizing Ehrlich’s reagent (employing ethyl alcohol instead of isoamyl alcohol) is employed.
Principle of Ehrlich’s Indole Test: Tryptophane, an amino acid, can undergo deamination and hydrolysis by bacteria that express the enzyme tryptophanase. The enzymatic action leads to the generation of indole through reductive deamination, with indolepyruvic acid serving as an intermediate molecule. Tryptophanase catalyzes the deamination process, where the amine (-NH2) group of the tryptophane molecule is removed. The end products of this reaction include indole, pyruvic acid, ammonium (NH4+), and energy. The presence of pyridoxal phosphate as a coenzyme is necessary for the enzymatic activity.

When indole is mixed with Kovac’s Reagent, consisting of hydrochloric acid, p-dimethylaminobenzaldehyde, and amyl alcohol, the solution undergoes a color change from yellow to cherry red. Since amyl alcohol is not soluble in water, the red coloration forms as an oily layer on top of the broth. In the spot test, indole reacts with p-Dimethylaminocinnamaldehyde (DMACA) in the filter paper matrix under acidic conditions, resulting in the formation of a blue to blue-green compound. This Indole Spot Reagent has been proven effective in detecting indole production among members of the Enterobacteriaceae family and certain anaerobic species.
Chemical Properties of Indole
In contrast to most amines, indole does not exhibit basic properties. The aromatic nature of the ring, similar to pyrrole, prevents the lone pair of electrons on the nitrogen atom from being available for protonation. However, strong acids like hydrochloric acid can protonate indole. Interestingly, indole is primarily protonated at the C3 position rather than N1 due to the enamine-like reactivity of the portion of the molecule located outside the benzene ring. The protonated form of indole has a pKa of -3.6. The sensitivity of many indolic compounds, including tryptamines, to acidic conditions is attributed to this protonation phenomenon.
In terms of reactivity, indole shares similarities with benzene but is generally more reactive. The lone pair of electrons on the nitrogen atom in indole contributes to an aromatic sextet, which hinders easy protonation and imparts a lack of basic properties. However, in the presence of strong bases, indole exhibits weak NH-acidic properties.
As a weak acid, indole can form N-sodium indole in a solution containing sodium in liquid ammonia (NH3), and N-potassium indole with the addition of potassium hydroxide (KOH) at a temperature of 130 °C. (Figure 6)
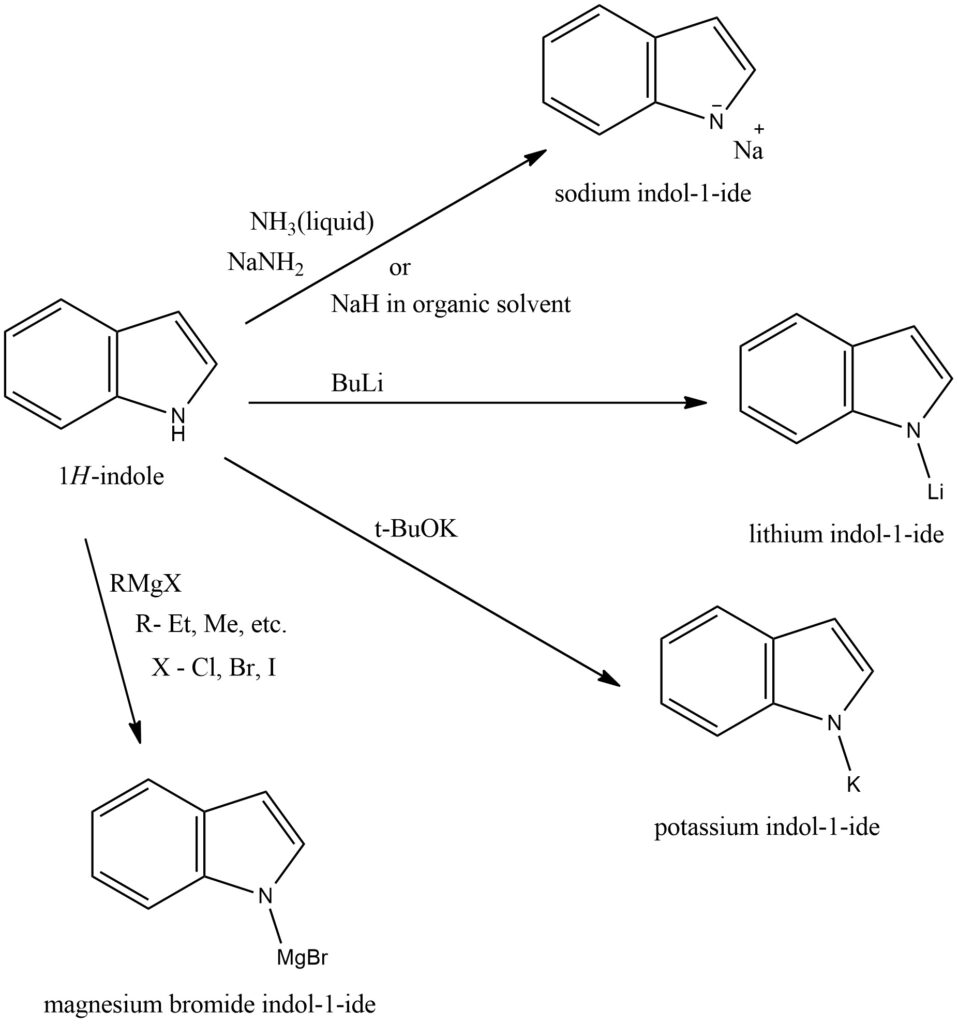
Acetylation of indole takes place at position 3 when using acetic acid, while in the presence of sodium acetate (CH3COONa), acetylation occurs at position 1. Acetic anhydride leads to the formation of 1,3-diacetylindole. Indole readily undergoes attachment to unsaturated ketones and nitriles through the alpha, beta double bond. It’s worth noting that indole exhibits acidophobic properties, meaning it has a tendency to avoid or resist interactions with acids. Figure 7.
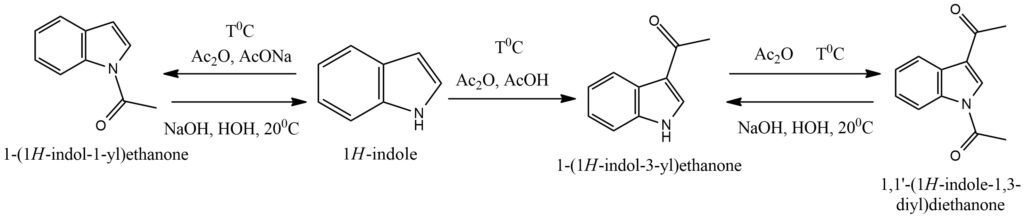
Indole exhibits aromatic characteristics due to its ring structure. Electrophilic substitution reactions primarily take place at the carbon atom located at position 3. Nitration of indole is commonly achieved using benzoyl nitrate as the reagent of choice. Figure 8.
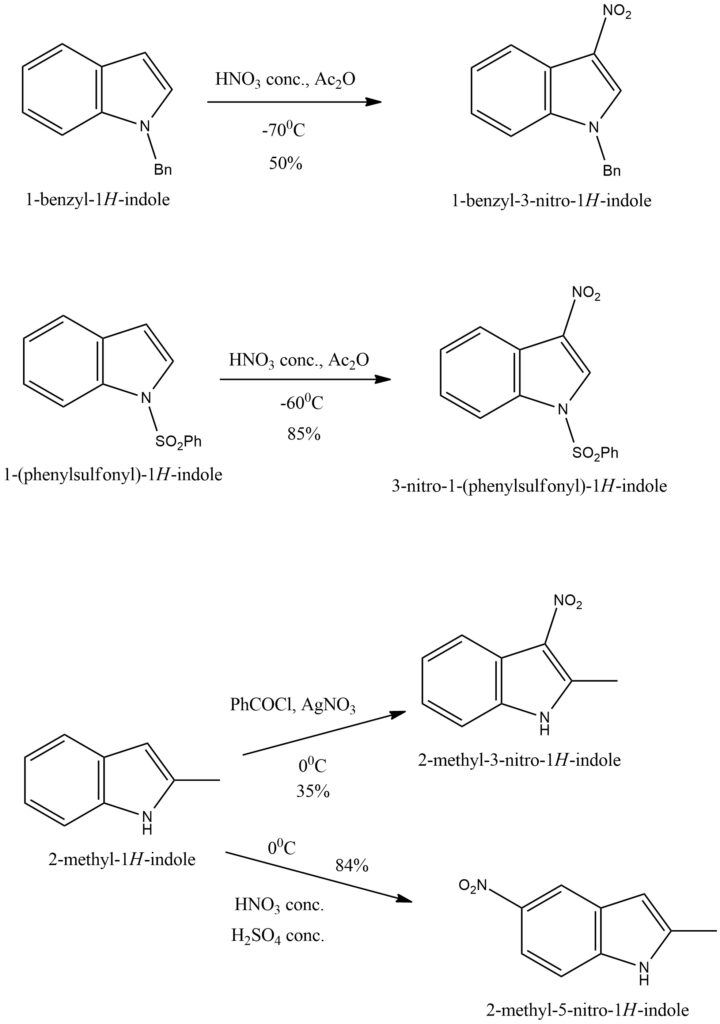
The bromination of indole using dioxane dibromide. Figure 9
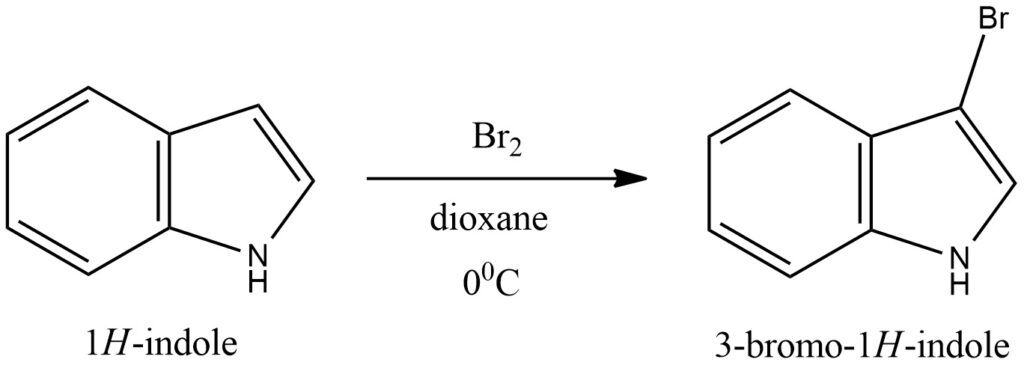
The chlorination of indole utilizing SO2Cl2. Figure 10
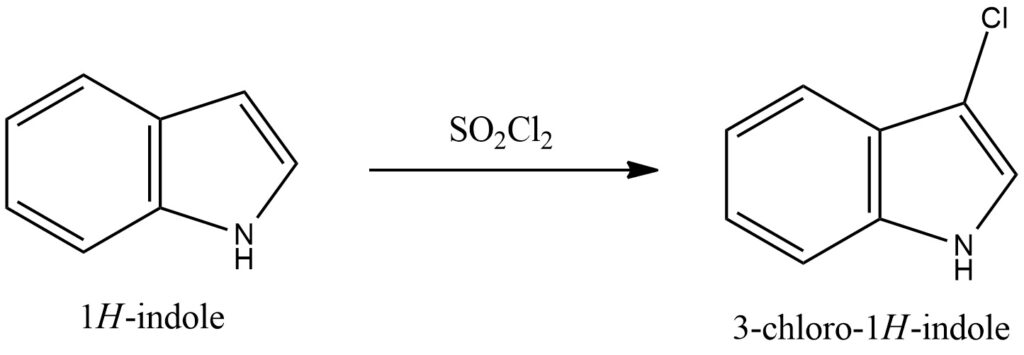
The alkylation of indole employing highly reactive alkyl halides. Figure 11

The aminomethylation of indole through the Mannich reaction. Figure 12
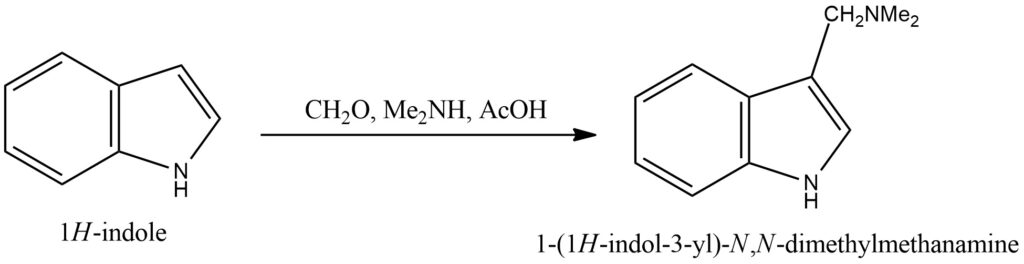
The Vilsmeier reaction involving indole with DMF (dimethylformamide) and POCl3 (phosphoryl chloride) is a synthetic method used to introduce a formyl group (CHO) onto the indole ring. In this reaction, DMF acts as a reactant and a solvent, while POCl3 serves as a reagent for the formylation process. The Vilsmeier reaction with indole, DMF, and POCl3 results in the formation of N-formylindole. This reaction is commonly employed in organic synthesis to modify the indole structure and create functionalized derivatives. Figure 13

In the process of hydrogenation, when indole is subjected to mild conditions, the reduction selectively occurs on the pyrrole ring. However, under more severe conditions, both the pyrrole and benzene rings undergo reduction. Figure 14
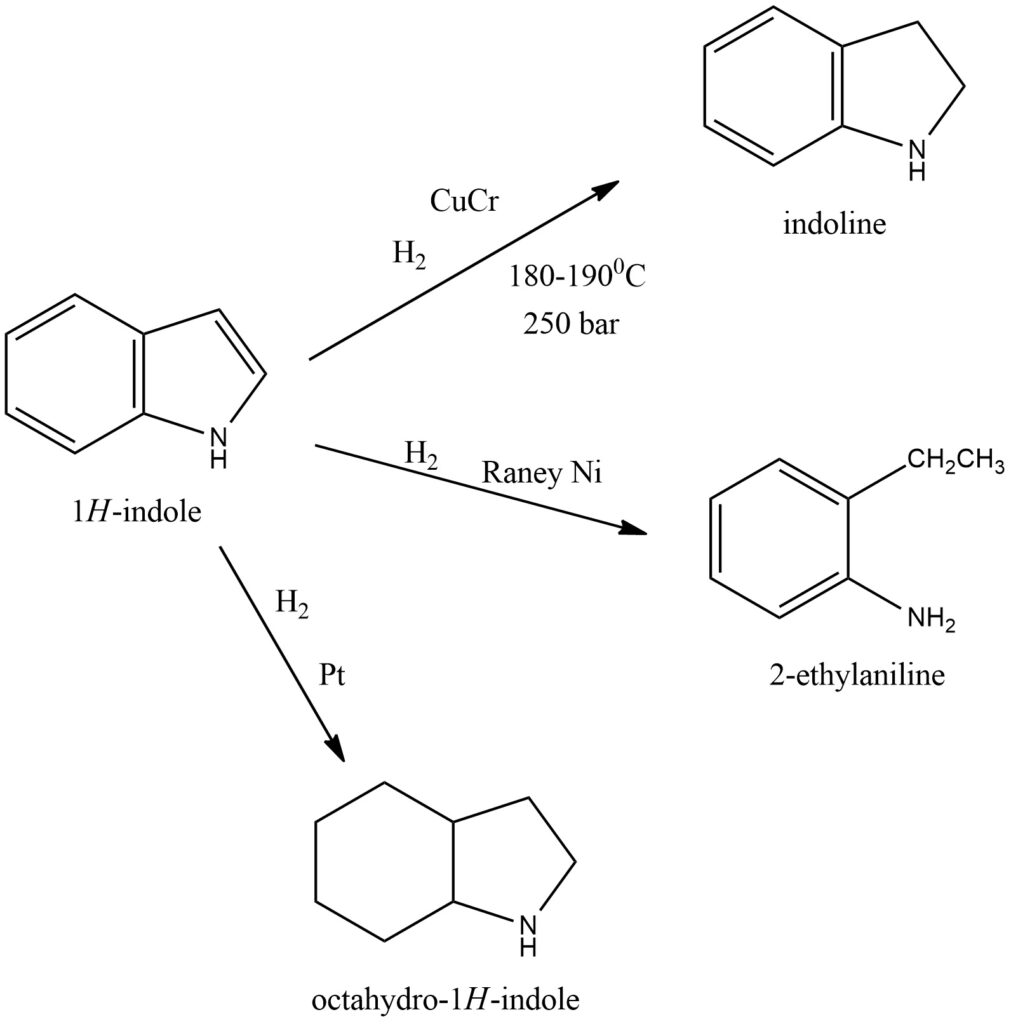
The dimerization of indole refers to the chemical reaction in which two indole molecules combine to form a dimeric compound. This reaction can occur through various methods, such as oxidative coupling or acid-catalyzed condensation. The resulting dimeric product can exhibit unique chemical properties and potentially serve as a building block for the synthesis of more complex indole derivatives. The dimerization of indole represents an important avenue in indole chemistry, providing opportunities for the creation of novel compounds with diverse applications in pharmaceuticals, materials science, and other fields. Figure 15
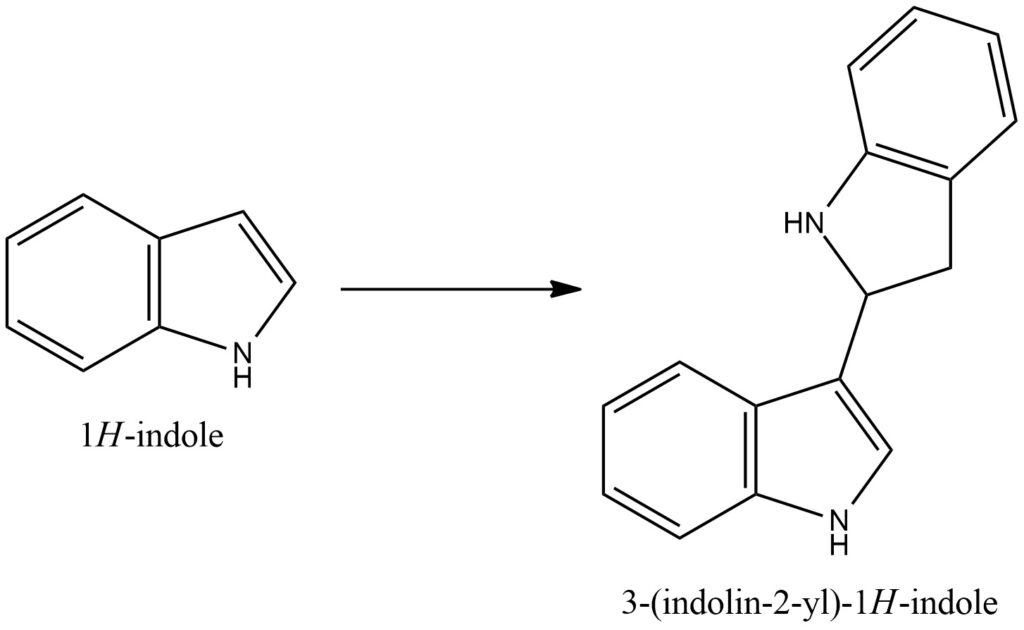
The azo coupling of indole involves the reaction of an indole compound with an aromatic diazonium salt, resulting in the formation of an azo compound. This reaction typically occurs under mild conditions and is facilitated by a suitable coupling agent or catalyst. The azo coupling of indole allows for the introduction of azo functional groups into the indole structure, leading to the formation of novel compounds with diverse properties. Figure 16
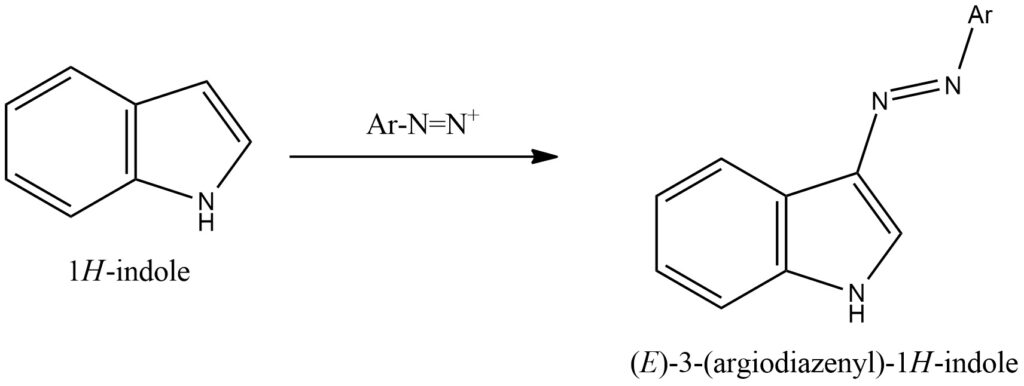
The oxidation of indoles utilizing potent oxidizing agents results in the cleavage of the pyrrole ring. This reaction leads to the fragmentation of the indole structure, resulting in the formation of distinct chemical species. The use of strong oxidizing agents facilitates the breaking of chemical bonds within the pyrrole ring, leading to the generation of diverse products with altered properties. Figure 17.
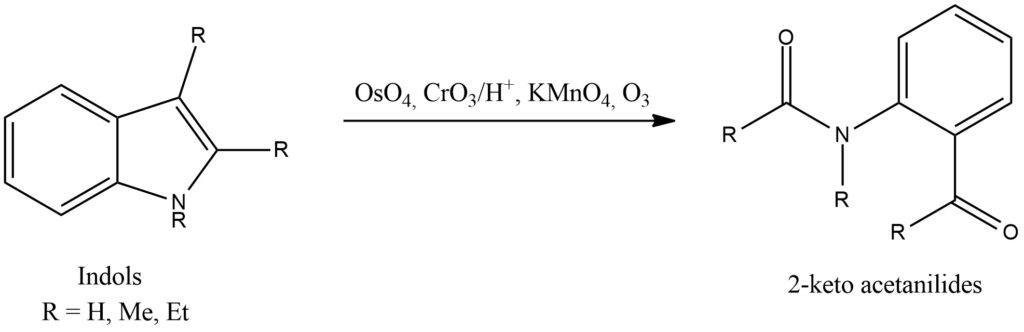
The sulfonation of indole using pyridine sulfotrioxide is a chemical reaction that introduces a sulfonic acid group (-SO3H) onto the indole molecule. Pyridine sulfotrioxide acts as a sulfonating agent, facilitating the addition of the sulfonic acid group to the indole ring. Figure 18
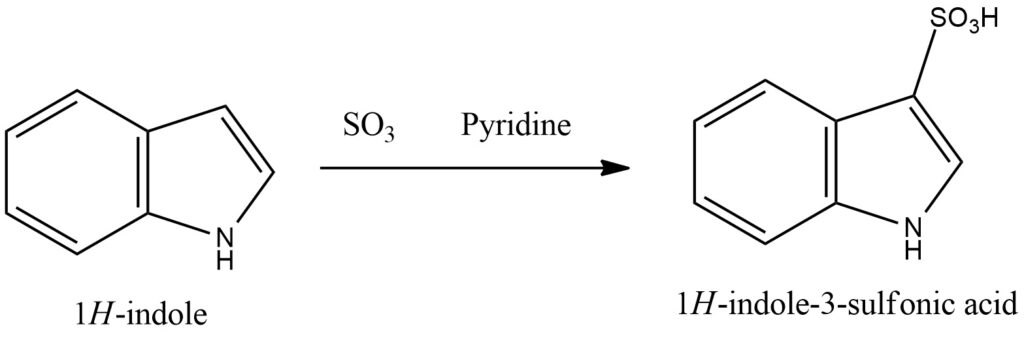
Synthesis of Indole [10-16]
Indole, along with its various derivatives, can be synthesized through a diverse array of methods. The primary pathways employed in industrial settings involve commencing with aniline and subjecting it to vapor-phase reactions with ethylene glycol, facilitated by catalysts. Typically, these reactions are carried out within the temperature range of 200 to 500 °C. Remarkably, the yields achieved can reach an impressive 60%. Additionally, other compounds such as formyltoluidine, 2-ethylaniline, and 2-(2-nitrophenyl)ethanol can serve as precursor materials for indole synthesis, undergoing cyclization reactions to yield the desired product. Figure 19
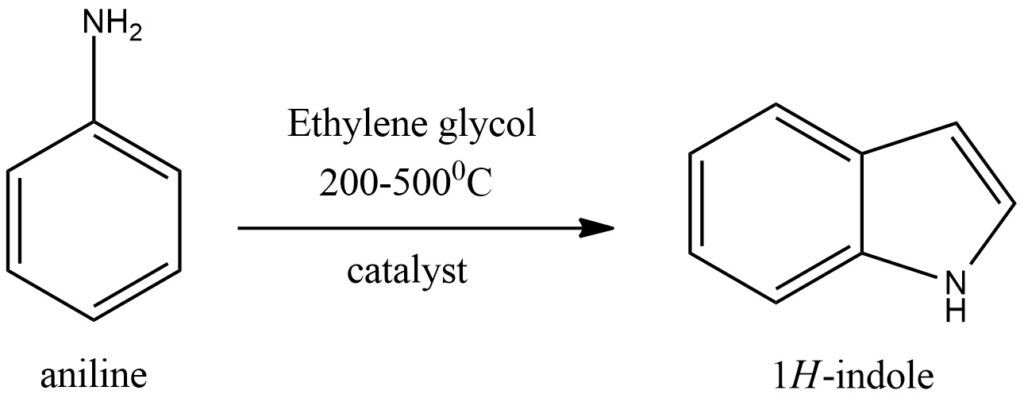
The Leimgruber–Batcho indole synthesis represents an efficient and reliable approach to the synthesis of indole and its substituted derivatives. Originally introduced in a patent publication back in 1976, this method stands out for its high-yielding nature and ability to generate substituted indole compounds. It holds particular significance within the pharmaceutical industry, as numerous pharmaceutical drugs incorporate specifically substituted indoles in their molecular structures. The Leimgruber–Batcho indole synthesis involves a series of organic reactions that transform o-nitrotoluenes into indole compounds. The initial step entails the formation of an enamine through the utilization of N,N-dimethylformamide dimethyl acetal and pyrrolidine. Subsequently, in the second step, the desired indole is produced through reductive cyclization. The reductive cyclization step can be achieved using various agents, including Raney nickel and hydrazine as illustrated in the provided scheme. Alternatively, palladium-on-carbon with hydrogen, stannous chloride, sodium hydrosulfite, or iron in acetic acid can also serve as effective reducing agents in this process. Figure 20
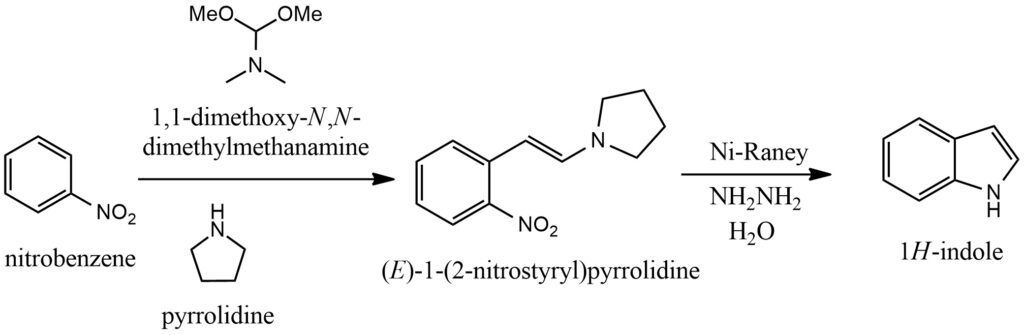
The Fischer indole synthesis is a notable chemical transformation that enables the production of the aromatic heterocycle known as indole. This reaction involves the interaction of a (substituted) phenylhydrazine with an aldehyde or ketone under acidic conditions. The discovery of this reaction is credited to Emil Fischer in 1883. Presently, the synthesis of antimigraine drugs belonging to the triptan class often employs the Fischer indole synthesis as a key step. Various catalysts can facilitate this reaction, including Brønsted acids such as HCl, H2SO4, polyphosphoric acid, and p-toluenesulfonic acid, as well as Lewis acids like boron trifluoride, zinc chloride, iron chloride, and aluminum chloride. These catalysts play a crucial role in promoting the conversion of the reactants into the desired indole compound. Figure 21
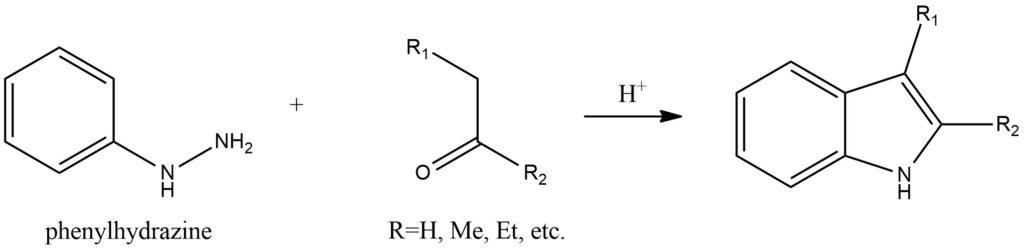
Conclusion
Indole is a versatile and significant heterocyclic compound that plays a crucial role in various fields, including pharmaceuticals and organic synthesis. Its synthesis can be achieved through several efficient methods, such as the Leimgruber-Batcho indole synthesis and the Fischer indole synthesis. These methods enable the production of indole and its substituted derivatives with high yields. The pharmaceutical industry particularly benefits from indole synthesis, as many drugs incorporate specifically substituted indole moieties for their desired biological activity. The reductive cyclization and enamine formation steps are key aspects of indole synthesis, and various catalysts, both Brønsted acids and Lewis acids, can be employed to facilitate the reactions. Overall, indole and its derivatives continue to be of significant interest in scientific research and drug development, showcasing their importance in the field of organic chemistry.
Bibliography
- https://pubchem.ncbi.nlm.nih.gov/compound/798
- https://www.chemspider.com/Chemical-Structure.776.html
- https://en.wikipedia.org/wiki/Indole
- https://pubchem.ncbi.nlm.nih.gov/source/hsdb/599#section=Body-Burden-%28Complete%29
- https://go.drugbank.com/drugs/DB04532
- International Union of Pure and Applied Chemistry. Nomenclature of Organic Chemistry: IUPAC Recommendations and Preferred Names 2013. The Royal Society of Chemistry. p. 213. doi:10.1039/9781849733069. ISBN 978-0-85404-182-4. https://books.rsc.org/books/monograph/180/Nomenclature-of-Organic-Chemistry
- Jin-Hyung Lee, Jintae Lee Indole as an intercellular signal in microbial communities. FEMS Microbiology Reviews, 2010, Volume 34, Issue 4, pp. 426–444, https://doi.org/10.1111/j.1574-6976.2009.00204.x https://academic.oup.com/femsre/article/34/4/426/538792?login=false
- Zhang L.S., Davies S.S. Microbial metabolism of dietary components to bioactive metabolites: opportunities for new therapeutic interventions. Genome Med., 2016, 8, 46. https://doi.org/10.1186/s13073-016-0296-x https://genomemedicine.biomedcentral.com/articles/10.1186/s13073-016-0296-x#citeas
- https://microbiologyinfo.com/indole-test-principle-reagents-procedure-result-interpretation-and-limitations/
- Gribble G. W. Recent developments in indole ring synthesis—methodology and applications. J. Chem. Soc. Perkin Trans., 2000, 1, 7, p. 1045. DOI https://doi.org/10.1039/A909834H https://pubs.rsc.org/en/content/articlelanding/2000/P1/a909834h
- Cacchi S., Fabrizi G. Synthesis and Functionalization of Indoles Through Palladium-catalyzed Reactions. Chem. Rev., 2005, 105, 7, pp. 2873–2920. https://doi.org/10.1021/cr040639b https://pubs.acs.org/doi/10.1021/cr040639b
- Humphrey G. R., Kuethe J. T. Practical Methodologies for the Synthesis of Indoles. Chem. Rev., 2006, 106, 7, pp. 2875–2911. https://doi.org/10.1021/cr0505270 https://pubs.acs.org/doi/10.1021/cr0505270
- Collin, Gerd; Höke, Hartmut. “Indole”. Ullmann’s Encyclopedia of Industrial Chemistry. Weinheim: Wiley-VCH. doi:10.1002/14356007.a14_167
- https://en.wikipedia.org/wiki/Leimgruber%E2%80%93Batcho_indole_synthesis
- https://en.wikipedia.org/wiki/Fischer_indole_synthesis
- https://en.wikipedia.org/wiki/Br%C3%B8nsted%E2%80%93Lowry_acid%E2%80%93base_theory