- The process of creating a drug - May 23, 2023
- How do scientists model mental disorders in animals? - May 23, 2023
Abstract
2-Iodo-4′-Methylpropiophenone stands as an new exemplary member within the esteemed haloketone classification. Acknowledged as iodoketone-4, this compound serves as an essential building block for the production of mephedrone. This publication delivers an all-encompassing account of 2-Iodo-4′-Methylpropiophenone, encompassing its fundamental particulars, physico-chemical attributes, chemical insights, synthetic procedures, conclusive observations, and reference sources.
https://www.youtube.com/watch?v=U3HZsSe1tNs
General Information About 2-Iodo-4′-Methylpropiophenone [1-4]
Other synonyms names of 2-Iodo-4′-Methylpropiophenone are: 4′-Methyl-alpha-iodopropiophenone; 2-Iodo-1-(4-methylphenyl)-1-propanone; 2-Iodo-1-(p-tolyl)propan-1-one; 2-Iod-1-(4-methylphenyl)-1-propanon; 2-Iodo-1-(4-methylphenyl)-1-propanone.
IUPAC Name of 2-Iodo-4′-Methylpropiophenone: 2-iodo-1-(4-methylphenyl)propan-1-one
CAS number is 236117-38-7
Trade names are Iodoketone-4; Iodketone-4
Different analogs of Iodoketones. This Analogs of Iodoketones are presented on Figure 1

Figure 1. Analogs of Iodoketones
Physico-Chemical Properties of 2-Iodo-4′-Methylpropiophenone [1-4]
- Molecular Formula C10H11IO
- Molar Weight 274.1 g/mol
- Boiling point 310.5±25.0 °C at 760 mmHg ℃
- Melting Point N/A
- Color/Form: Pale yellow powder
Structural formula present on Figure 2.
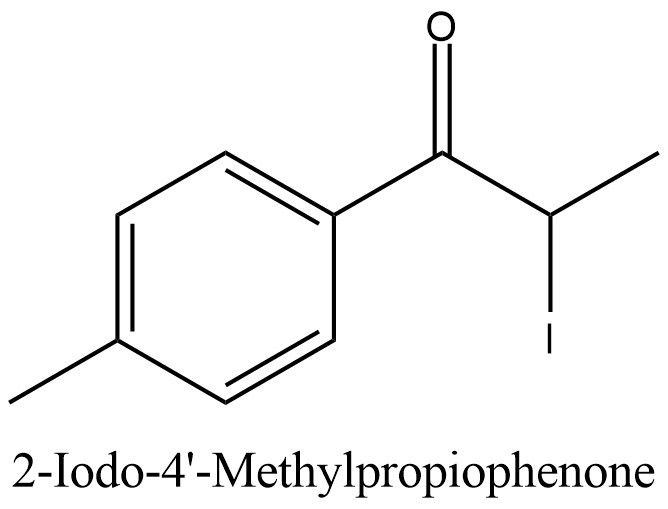
Powder and crystalline solid possible of the 2-Iodo-4′-Methylpropiophenone can be seen in the picture provided in Figure 3.

Commercial 2-Iodo-4′-Methylpropiophenone can be seen in the picture provided in Figure 4.
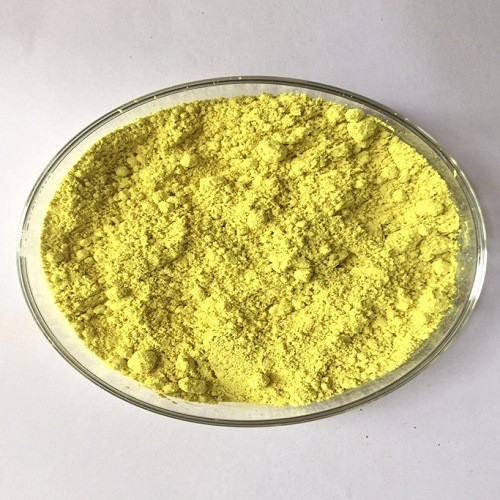
Chemical Information of 2-Iodo-4′-Methylpropiophenone [1-5]
The compound known as 2-Iodo-4′-Methylpropiophenone exhibits a distinctive arrangement with an iodine atom located at position 2 and a methyl group (CH3) attached to the aromatic ring at the 4′-position. Propiophenone derivatives, renowned for their versatility, find extensive employment in the realm of organic synthesis. They are valued as crucial starting materials for the production of diverse organic compounds through various synthetic pathways.
Chemical reactions
The rearrangement known as the Favorskii reaction is a chemical process that entails the reorganization of carbonyl compounds containing α-halo groups, typically in the presence of a potent base. This reaction induces the migration of the α-halo group to a different position within the molecule, leading to the formation of a distinct compound. The Favorskii rearrangement possesses extensive applications in the realm of organic synthesis, serving as a means to generate intricate molecules with modified carbon frameworks. It facilitates the introduction of novel functional groups and the creation of strained ring systems. The reaction progresses via the formation of a carbanion intermediate, which subsequently undergoes rearrangement to yield the ultimate product. An illustrative instance of the Favorskii rearrangement involves the conversion of α-halo ketones into α-carbonyl carbanions, followed by rearrangement to produce carboxylic acids or their derivatives. This reaction has found widespread utilization in the synthesis of natural compounds, pharmaceuticals, and other valuable substances. It is crucial to note that the Favorskii rearrangement necessitates meticulous control of reaction conditions, as the outcome can be remarkably influenced by the choice of base, temperature, and reaction duration. Optimizing these parameters is of paramount importance to obtain the desired product with enhanced selectivity and yield. Figure 5.
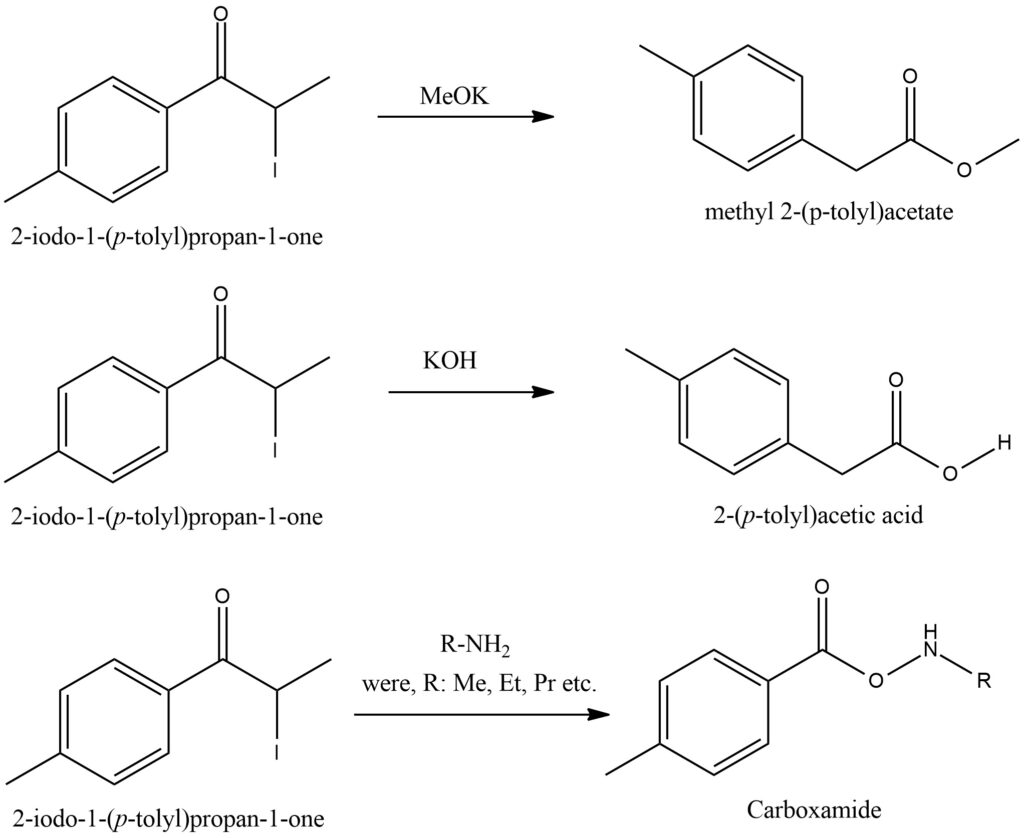
The Grignard reaction is a fundamental organic reaction that involves the reaction of a Grignard reagent with a ketone compound. Grignard reagents are organometallic compounds typically formed from alkyl or aryl halides and magnesium metal in the presence of an ether solvent. In the Grignard reaction with ketones, the Grignard reagent acts as a nucleophile and attacks the electrophilic carbon of the ketone’s carbonyl group. This initiates the formation of a carbon-carbon bond between the carbon of the Grignard reagent and the carbonyl carbon of the ketone. As a result, a new carbon-carbon bond is formed, and an alkoxide intermediate is generated. The alkoxide intermediate formed is unstable and readily reacts with water or an acid source to yield the final product. Hydrolysis with water produces an alcohol, while reaction with an acid source leads to the formation of a corresponding carboxylic acid. The Grignard reaction with ketones is widely utilized in organic synthesis for the introduction of new functional groups and the formation of complex carbon frameworks. It offers a versatile and efficient method for the construction of various organic compounds, including alcohols, carboxylic acids, and other derivatives. Care must be taken in handling Grignard reagents, as they are air and moisture sensitive, and reactions should be conducted under anhydrous conditions. Figure 6.
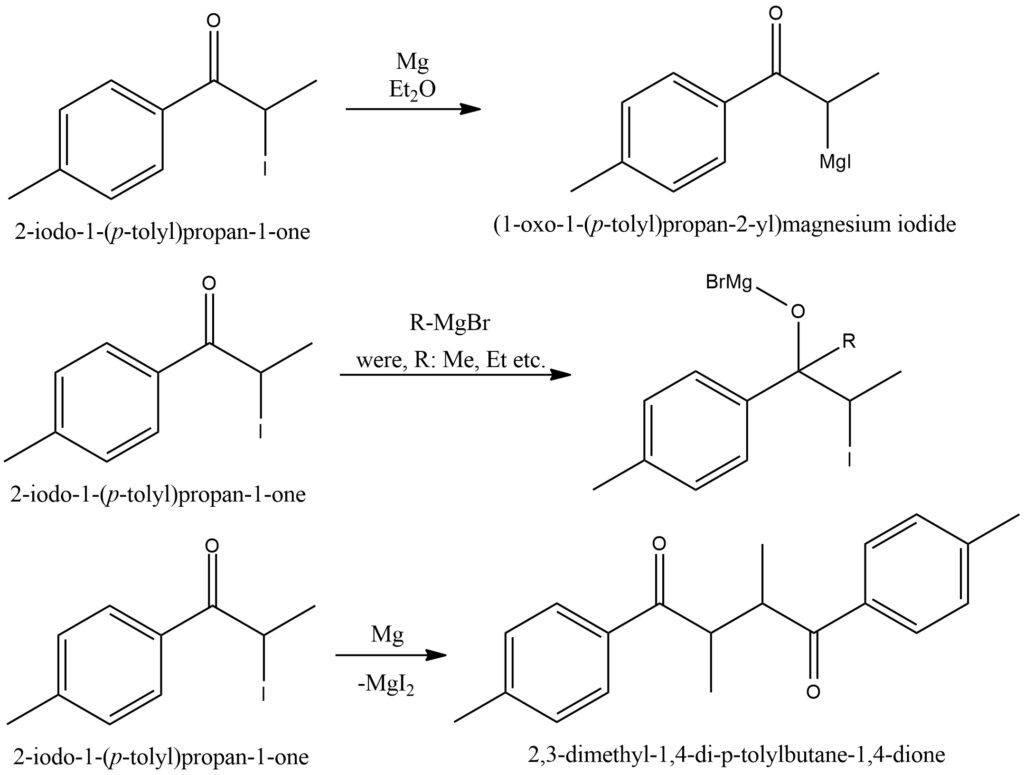
The Perkow reaction, also referred to as the Perkow synthesis, is a chemical process that involves the conversion of a ketone compound and an organic trivalent phosphorus derivative. In the Perkow reaction, a ketone reacts with an organic trivalent phosphorus compound, such as allyl phosphine or phenyl phosphine, in the presence of an acid or base catalyst. The reaction proceeds by forming an intermediate complex, where the phosphorus atom establishes a bond with the carbonyl oxygen of the ketone. Subsequently, electron redistribution takes place, leading to the creation of a new bond between the carbonyl oxygen and phosphorus. Depending on the specific ketone and trivalent phosphorus compound employed, the Perkow reaction can yield diverse heterocyclic compounds with varied structures. Figure 7.

The Darzen condensation, also referred to as the Darzen glycidic ester condensation, is a chemical reaction that involves the condensation of an α,β-unsaturated acid chloride or anhydride with an alkoxide or phenoxide nucleophile. This reaction was initially discovered by Victor Darzen, a Swiss chemist, during the early 20th century. In the Darzen condensation, the acid chloride or anhydride undergoes a reaction with the nucleophile, resulting in the formation of a β-hydroxy ester or β-hydroxy ketone. The reaction proceeds by the nucleophile attacking the electrophilic carbonyl carbon of the acid chloride or anhydride, leading to the elimination of a chloride ion or anhydride molecule. This step gives rise to a cyclic intermediate, which subsequently undergoes intramolecular nucleophilic attack by the alkoxide or phenoxide group. As a result, the desired β-hydroxy ester or β-hydroxy ketone is formed. The Darzen condensation serves as a versatile method for synthesizing β-hydroxy esters and β-hydroxy ketones. These compounds are essential building blocks in organic synthesis and can be further modified into various functional groups. The reaction can be conducted under mild conditions and provides good control over regioselectivity and stereochemistry. Figure 8.
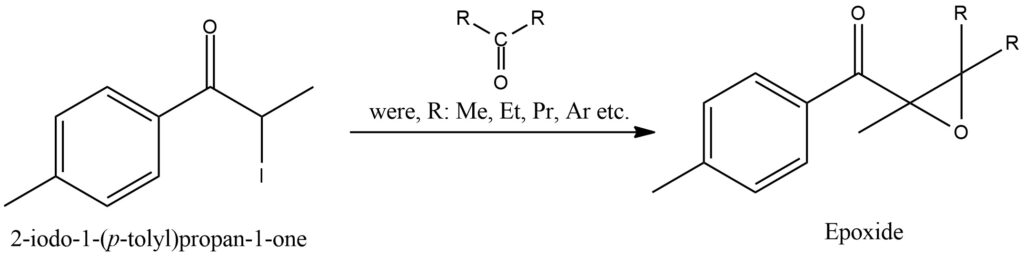
Ketone hydrogenation, also known as ketone reduction, is a chemical process that involves the addition of hydrogen (H2) to a ketone functional group, resulting in the formation of an alcohol. This reaction is widely utilized in organic synthesis and commonly relies on transition metal catalysts like palladium, platinum, or nickel. In ketone hydrogenation, the ketone molecule undergoes a reduction process in the presence of a hydrogen source and a suitable catalyst. Molecular hydrogen (H2) gas serves as the typical hydrogen source, and the catalyst facilitates the activation of hydrogen and its subsequent addition across the ketone carbonyl group. Consequently, the ketone is transformed into an alcohol, introducing a new hydrogen atom. Ketone hydrogenation is a versatile and valuable transformation in organic chemistry, as it enables the selective reduction of ketones to alcohols while leaving other functional groups within the molecule unaltered. The reaction conditions, including temperature, pressure, catalyst, and solvent, can be adjusted to control the selectivity and stereochemistry of the hydrogenation process. Hydrogenation of ketones finds applications in various fields, including pharmaceutical synthesis, fine chemicals production, and the derivation of natural products. It facilitates the conversion of carbonyl groups into more versatile and functional alcohol moieties that can be further manipulated in subsequent chemical transformations. Figure 9.
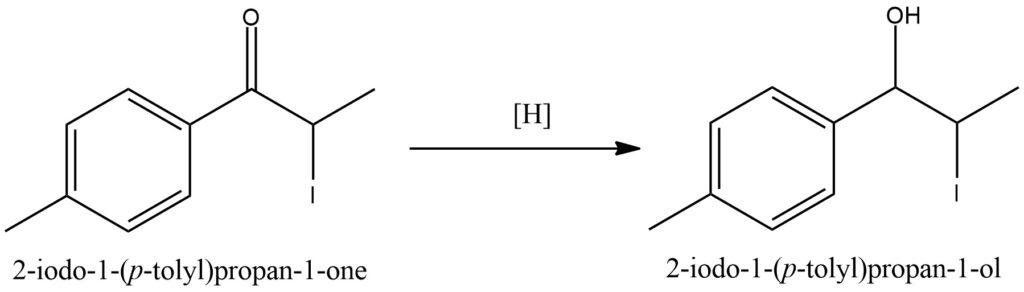
Ketones undergo a reaction with hydroxylamine to form ketoximes while releasing water as a byproduct. This chemical transformation, known as ketoximation, entails the addition of hydroxylamine (NH2OH) to the ketone’s carbonyl group, resulting in the formation of a C=N bond and the conversion of the carbonyl oxygen into a hydroxyl group. During ketoximation, the lone pair of electrons on the nitrogen atom of hydroxylamine attacks the electrophilic carbon atom of the ketone’s carbonyl group. This interaction gives rise to an intermediate species where the oxygen of the carbonyl group forms a bond with the nitrogen atom. Subsequently, water is eliminated from the intermediate, leading to the formation of the ketoxime product. Ketoximes are characterized by the presence of the C=N bond, which imparts distinct chemical properties to these compounds. They serve as versatile intermediates in various organic transformations, including the synthesis of amides, oximes, and other functional groups. These reactions allow for the further modification and diversification of ketoximes into a wide range of valuable organic compounds. Figure 10.
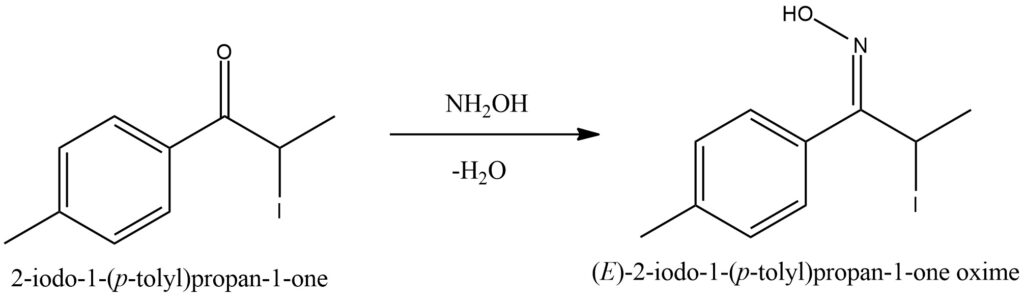
When hydrazine and ketones undergo a chemical reaction, the resulting products can be either hydrazones or azines, depending on the specific reaction conditions. The proportion of hydrazine to ketone plays a crucial role in determining the final outcome: a 1:1 ratio yields hydrazones, while a 1:2 ratio produces azines. For the formation of hydrazones, one molecule of hydrazine reacts with one molecule of ketone. During this process, the nitrogen atoms in hydrazine attack the carbonyl carbon in the ketone molecule. This leads to the creation of a C=N double bond and the transformation of the carbonyl oxygen into a hydrazone group. On the other hand, azines are formed when two molecules of hydrazine react with one molecule of ketone. Both nitrogen atoms in hydrazine actively participate in the reaction, resulting in the formation of a C=N double bond within the ketone molecule. Additionally, the carbonyl oxygen undergoes conversion into an azine group. Figure 11.
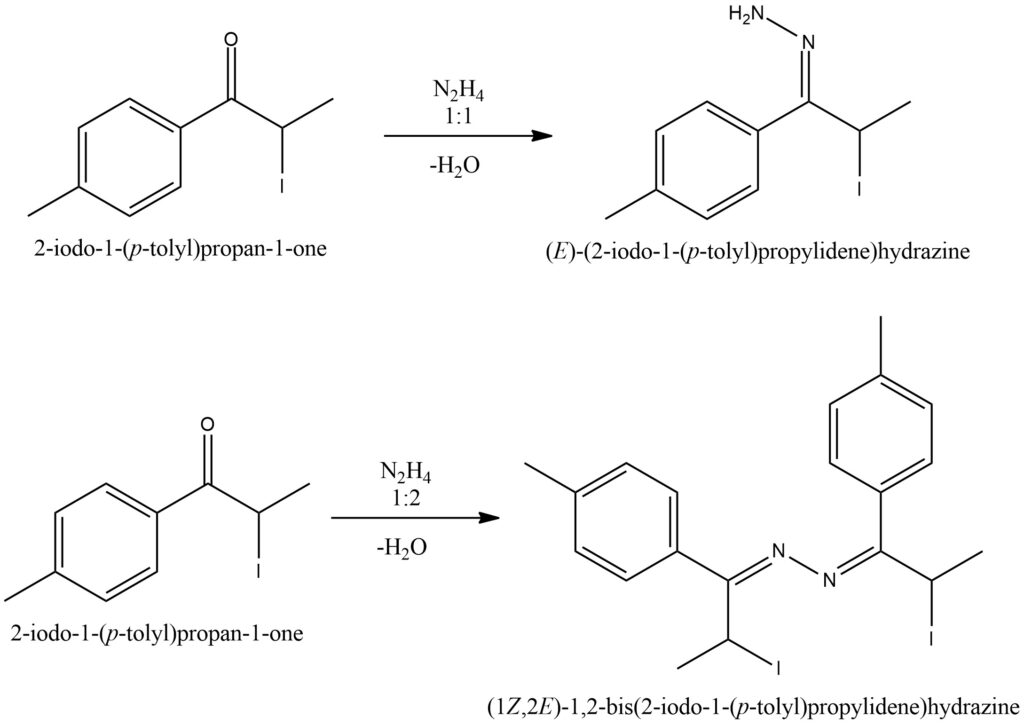
The Leuckart-Wallach reaction is a chemical process that facilitates the transformation of a carbonyl compound, which can be a ketone or aldehyde, into an amine. This reaction is widely utilized in the synthesis of primary and secondary amines. In the Leuckart-Wallach reaction, the carbonyl compound reacts with formic acid (HCOOH) and an amine, typically ammonium formate (NH4HCO2), at elevated temperatures. The reaction progresses through several stages, beginning with the formation of an imine intermediate, followed by subsequent reduction and rearrangement processes. Ultimately, the carbonyl group undergoes conversion into an amine group. It is important to note that the selectivity and efficiency of the Leuckart-Wallach reaction can be influenced by various reaction conditions, including temperature, reaction time, and the choice of catalyst. To enhance the reaction rate and yield, appropriate catalysts such as Raney nickel or platinum on carbon are often employed. These catalysts play a crucial role in facilitating the reaction, making it more favorable and efficient in terms of both speed and product formation. Figure 12.
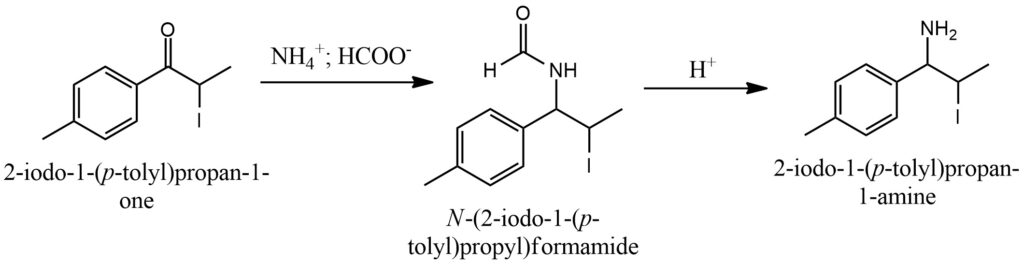
Mephedrone Synthesis Figure 13.
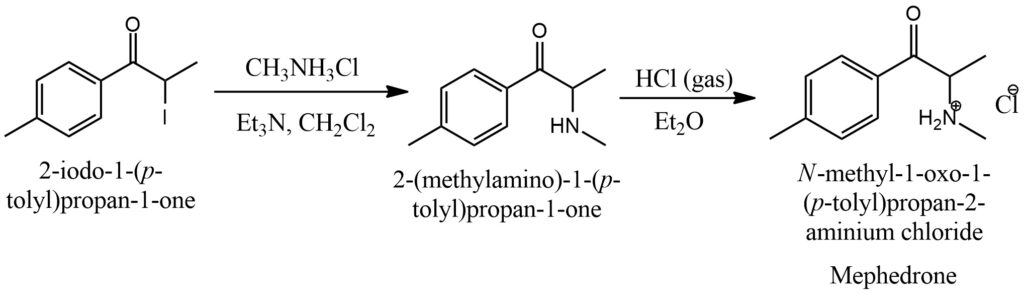
Synthesis of 2-Iodo-4′-methylpropiophenone [6]
In a reactor, a mixture of 4′-Methylpropiophenone and IPA is prepared. Subsequently, Copper(II) oxide and Iodine are added to the mixture. The reaction mixture is then heated to a temperature of 70 °C and left to react for 24 hours while being continuously stirred and heated. Once the reaction time elapses, the stirrer is turned off, and the solution is allowed to settle to facilitate the precipitation of copper. After the copper precipitation, the heating process is continued. The resulting solution containing 2-Iodo-4′-methylpropiophenone is decanted from the precipitate, carefully pouring it out from the top of the reactor. The solution is then transferred to a freezer and left there for a period of 24 hours to promote crystal formation. The crystals of 2-Iodo-4′-methylpropiophenone are subsequently filtered using a nutsche filter. Cold IPA is used to wash the crystals while they are on the filter. Following the filtration, approximately two-thirds of the filtered solution is distilled off using a rotovap. The remaining solution is then subjected to a crystallization process and undergoes another washing step. Finally, the crystals of 2-Iodo-4′-methylpropiophenone are dried either under vacuum conditions or by allowing them to air dry. The depicted scheme showcases the operational flow outlined in Figure 14.
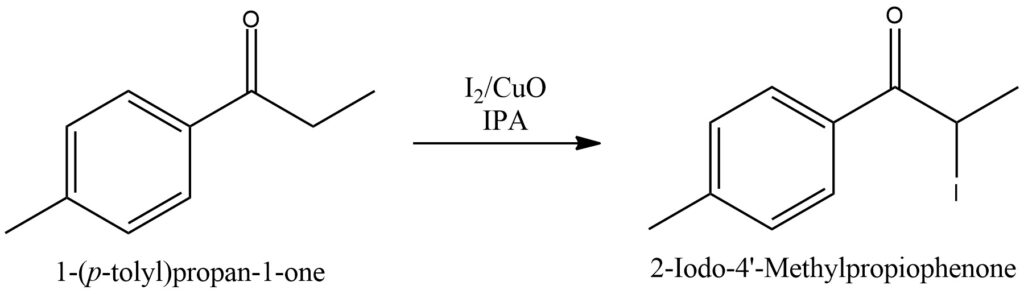
Conclusion
2-Iodo-4′-Methylpropiophenone represents a recent addition to the class of haloketones, exhibiting a unique combination of halogen-containing compounds and ketones properties. Widely employed in organic synthesis, this compound serves as a crucial building block for the production of mephedrone. The synthesis of 2-Iodo-4′-Methylpropiophenone involves the iodination process of its corresponding 4′-methylpropiophenone precursor. Notably, this compound is readily accessible in the market under the commercial designation of Iodketone-4. Furthermore, its distinctive chemical structure and versatile reactivity make it a valuable tool in various research and industrial applications.
Bibliography
- https://www.chemspider.com/Chemical-Structure.114989382.html?rid=056ff4a9-9999-4774-b536-66e36a674114
- https://pubchem.ncbi.nlm.nih.gov/compound/129960950
- https://pubchem.ncbi.nlm.nih.gov/compound/100979641#section=Structures
- https://www.amadischem.com/proen/619300/
- https://en.wikipedia.org/wiki/Ketone
- https://bbgate.com/threads/4-methylpropiophenone-iodination-to-2-iodo-4-methylpropiophenone-by-iodine-cooper-ii-oxide-video-manual.218/